CAR-T cell therapy, a groundbreaking advancement in the pharmaceutical industry over the last 20 years, exemplifies the innovative strides made in areas like recombinant proteins, mRNA technologies, and advanced cell therapies. This progress was supported by the European Regulation 1394/2007, which established the regulatory framework for advanced therapy medicinal products (ATMPs) in Europe, categorizing them into 4 product classes:
- Somatic Cell therapy medicinal products (sCTMP)
- Gene therapy medicinal products (GTMP)
- Tissue engineered products (TEP)
- Combined ATMPs
A notable development among these is the chimeric antigen receptor (CAR) T-cell therapy. CAR-T cells are classified under the gene therapy medicinal products (GTMP) category, as they are based on genetically modified T cells. The modification involves integrating a transgene into the T cell genome, coding for the CAR. This gene alteration produces CAR T-cells, which play a crucial role in the therapy’s immunological action.
The journey of CAR-T cell therapies began in the late 1980s. Despite a substantial pipeline and numerous ongoing clinical trials, only two autologous anti-CD19 CAR-T therapies have received marketing authorization in Europe and the United States so far:
- Kymriah®, tisangenlecleucel (Novartis Europharm), approved for the treatment of pediatric and young patients up to and including 25 years of age with B-cell acute lymphoblastic leukemia (ALL) that is refractory, in relapse post-transplant, or in second or later relapse and for adult patients with relapsed or refractory diffuse large B-cell lymphoma (DLBCL) after two or more lines of systemic therapy.
- Yescarta®, axicabtagene ciloleucel (Kite Pharma), approved for adult patients with relapsed or refractory DLBCL and primary mediastinal large B-cell lymphoma (PMBCL), after two or more lines of systemic therapy.
Currently, these therapies primarily target hematological (blood) cancers. In this blog post, we will delve into the key elements of CAR-T cell therapy, including the T-cell and the CAR, explore its regulatory framework and manufacturing processes, and discuss the future outlook for this immune effector cell (IEC) therapy.
CAR-T cell therapy: harnessing the power of T-cells
On the origins of B-cells and T-cells
T cells, also called T lymphocytes, are one of the most important components of the adaptive immune system, in addition to B cells.
All cellular elements of the blood, such as red blood cells, platelets and white blood cells, originate from the same precursor cells (also called progenitor cells), namely the hematopoietic stem cells in the bone marrow. These multipotent stem cells can differentiate into myeloid progenitor cells and lymphoid progenitor cells.
Monocytes (macrophage in mature form), dendritic cells, granulocytes and mast cells are derived from these myeloid progenitor cells. These cells will not be further discussed in this blog post. The second group of progenitor cells – the lymphoid progenitor cells – give rise to the lymphocytes and Natural killer cells (NK cells).
There are two main types of lymphocytes: B lymphocytes (B cells) and T lymphocytes (T cells). B cells mature in the bone marrow, while T cells migrate to the thymus to mature. Once matured, T and B cells enter the bloodstream and migrate to the peripheral lymphoid organs (e.g., the lymphoid tissues associated with mucosa such as the appendix, lymph nodes, spleen) to perform their adaptive immune response tasks.
These different lymphocytes cannot be distinguished based on morphological aspects, but can be classified based on their unique receptors, T cells and B cells, or the lack of a specific receptor in the case of NK cells.
3 specific types of T cells: starting materials for CAR-T cell therapy
The specific types of T cells obtained by leukapheresis (which will be described later) will serve as starting materials in the CAR-T manufacturing process, as explained further. Three main T-cell subsets can be distinguished, namely:
Effector T cells (Teff)
The effector T cells include the cytotoxic T cells (Tc-cells, also called CD8+ cells) and helper T cells (Th cells, also called CD4+cells)
- Th cells will assist the immune response by releasing cytokines.
- TC cells on the other hand are involved in the destruction of virally infected cells and tumor cells.
Regulatory T cells (Treg)
Regulatory T cells are CD4+ and CD25+ play a crucial role in the ceasing of the T-cell mediated immunity and therefore these cells are able to control and suppress the immune response.
Memory T cells (Tm)
Antigen-specific T cells that remain in the body after infection (immunological memory).
From naive T cells to effector T cells
After full maturation, the naive T cell (inactivated T cell) will screen for foreign antigens.
The T-cell antigen receptor (TCR) present in the membrane of the T cell recognizes peptides derived from foreign proteins or pathogens, for example, tumor cells or virus-infected cells, associated with a Major Histocompatibility Complex (MHC) molecule.
Four other signaling domains – collectively called CD3 – and the ζ chains, are required to let the T cell know that the peptide is bound.
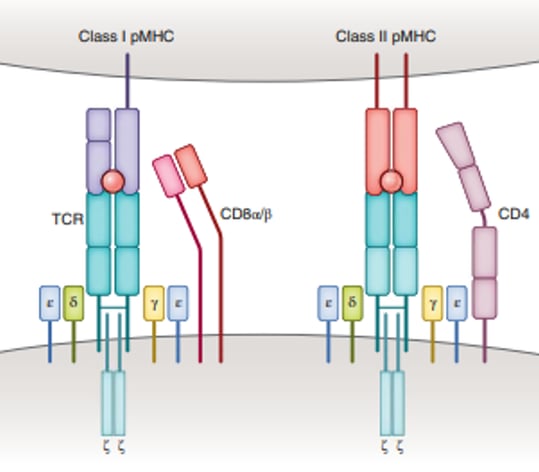
Figure 1 – Molecular basis of TCR-peptide MHC recognition. The TCR complex contains CD3ζ, CD3γ, CD3δ and CD3ε chains (source reference 3)
Depending on the MHC class (I or II), a different type of naive T cell will bind. Tc cells (CD8+) will recognize antigens in association with MHC class I molecules and Th cells are involved for MHC class II molecules (Figure 1).
For the proliferation and differentiation of the naive T cells into the effector T cells (activated T cells), a non-specific costimulatory signal must be received from the antigen-presenting cell (APC) in addition to the specific TCR binding. Indeed, CD28 of the T cell binds with CD80/CD86 on the APC (Figure 2).
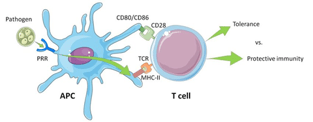
Figure 2 – Illustration of the activation of a T cell by the interaction between the antigen-presenting cell’s MHC Class II molecules and the T cell receptor (TCR) and costimulatory signal of the CD28 with CD80/CD86. (Source reference 4)
Although T cells are very effective, some pathogens or malignant cells have found ways to escape this antigen recognition and therefore can bypass the T cell surveillance system of the immune system. New technologies were invented to overcome this barrier, namely re-engineering the T cell into a CAR-T cell for CAR-T cell therapies.
CARs: genetically engineered antigen recognition
What are CARs?
CAR stands for Chimeric Antigen Receptor, as it is composed of several domains derived from different components through an engineered ex-vivo manipulation, such as chimeric antibodies (Ab) derived from different species (e.g., a combined human and mouse antibody).
In the case of CAR, the antigen recognition domain is derived from an Ab and the CD3ζ T-cell signaling domain is derived from the TCR.
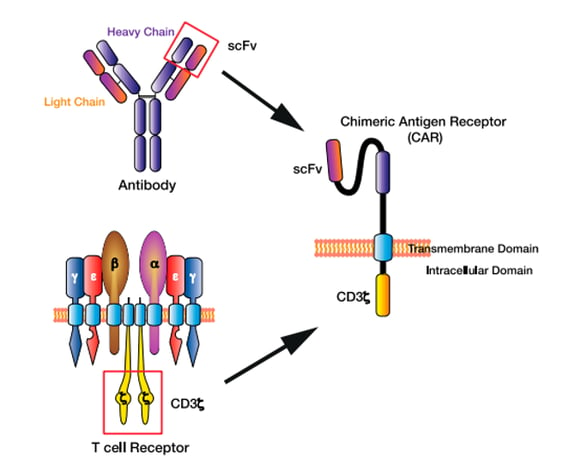
Figure 3 – The chimeric antigen receptor (CAR) T cell design. The single-chain variable fragment (scFv) of the CAR derived from the heavy and light chains of the antibody variable region, while the CAR CD3ζ domain is derived from the T cell receptor intracellular signalling domains. (source reference 5)
4 main CAR domains
Since the development of the first CAR, several new generations have been created to increase the persistence, activity, and regulation of CAR-T cells. CARs generally consist of 4 main domains with their specific actions:
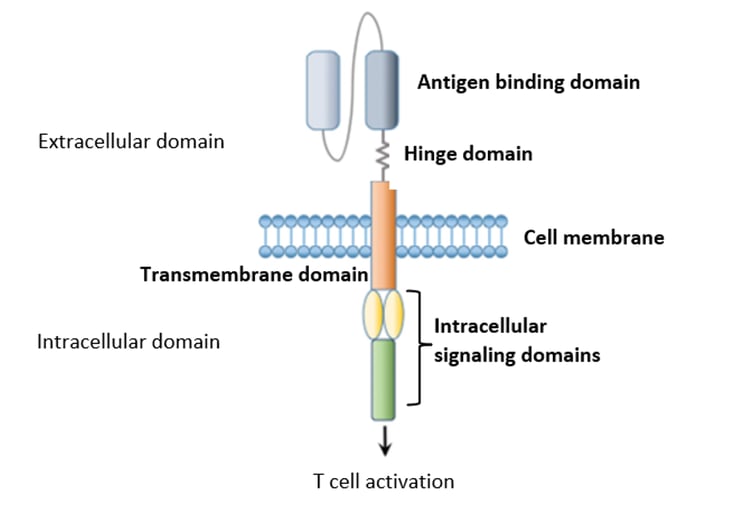
Figure 4 – CAR structure (source reference 7)
Antigen-binding domain
Specific binding of T cells to the target antigen in an MHC-independent manner.
Hinge domain
Facilitates access to the target Ag.
Transmembrane domain
Anchoring the CAR in the cell membrane (influences the stability and function of the CAR).
The intracellular signaling domain(s)
Typically contains the activation domain (CD3ζ) and one (or more) co-stimulatory domains (CD28, 4-1BB, ….). Different generations of CARs are defined based on the design of their signaling domains6.
The currently approved CAR-T cell therapies (Kymriah® and Yescarta®) both contain second-generation CARs. By developing the T-cell into a CAR-T cell, the T cell itself can attack specific targeted tumor cells and use its own T-cell immune mechanisms to destroy the cell.
CAR-T cell therapy: manufacturing process from donor (blood sample) to administration (infusion)
Currently, only two autologous CAR-T have been granted marketing authorization. Therefore, key aspects of the manufacturing process of an autologous therapy will be highlighted.
The industry is developing automated closed or semi-closed manufacturing processes (e.g. Miltenyi CliniMACS Prodigy) to reduce the risk of product contamination and accelerate the vein-to-vein time, i.e., reduce the entire manufacturing time from point of donation to administration.
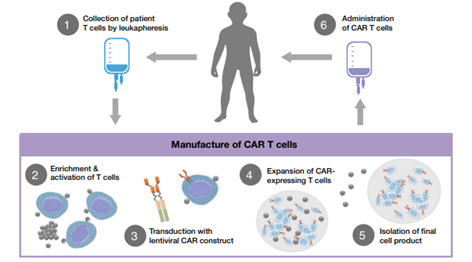
Figure 5 – Diagram of CAR T cell treatment process (source reference 8)
Step 1: Leukapheresis
The donation, procurement and testing of cells for ATMPs need to be executed according to the human tissues directive 2004/23/EC9 (Reg1394/2007 (14)).
Donors (patients) will undergo a leukapheresis. Leukapheresis is a specific separation technique in which the cellular components of the blood and more specifically the leukocytes (white blood cells) are collected. These peripheral blood mononuclear cells (PBMCs) contain the different lymphocytes (T cells/B cells/NK cells) and monocytes (macrophages, dendritic cells). Contaminants such as red blood cells and platelets can be removed by washing10. Washing and mononuclear (lymphocytes and monocytes) enrichment is performed by using devices such as the Lovo cell washer (Fresenius Kabi), the Terumo COBE 2991 (Terumo BCT) and the Sepax device (Sepax Technologies Inc.)10.
The medical condition of the autologous donor will affect the yield and purity of the CAR-T product. Therefore, donor eligibility criteria should be established prior to initiating CAR-T therapy and applicable procedures and standards should be applied to limit the variability of the material.
Step 2: T cell enrichment and activation
Figure 5 includes a purple box showing steps 2 to 5, as these steps must meet the requirement stated in EudraLex Volume 4 Part IV – GMP requirements for Advanced Therapy Medicinal Products11.
After leukapheresis and washing, T cell subsets (CD4, CD8, CD25 etc.) are enriched by using immunomagnetic separation on the Miltenyi CliniMACs and Prodigy devices)10. This step is performed by selecting CD4+, CD8+, CD25+ cells using magnetic beads coated with anti-CD4, anti-CD8, anti-CD25 antibodies. These processed T cells can either be used directly in further downstream processes or cryopreserved for later use.
In the next process step, T cells are activated, which is followed by the proliferation of T cells by adding specific cytokines. T-cell activation is obtained by simulating the in vivo simultaneous binding of the T-cell receptor CD3 and the co-receptor CD28 to their ligand, namely CD80/86 and MHC, which are represented in vitro by two antibodies anti-CD3 and anti-CD28 attached to an immunomagnetic bead (Dynal Dynabeads: Human TActivator) or Miltenyi beads (TransAct)10.
Other activation methods are also reported, for example, the use of Expamer (trademarked by Juno Therapeutics). Here, a soluble StrepTactin protein oligomer forms the backbone for the anti-CD3 and anti-CD28 ligands10.
Step 3 – 4: Transduction and expansion
Currently, the most commonly used devices are the G-Rex (Wilson Wolf Manufacturing), the Wave Bioreactor (GE Life Systems) and the CliniMACS Prodigy (Miltenyi Biotec)10. For the transduction of the T cells, mainly γ-retroviral vectors and lentiviral vectors are used that are added to the specific vessel in which the T cells are located. Other non-viral methods are also being investigated, such as transposon/transposase system or mRNA transfer via electroporation10.
Since these T-cells undergo genetic modification, CAR-T cell products must also comply with genetically modified organisms (GMO) requirements. This can be a major challenge as the GMO EU directives are implemented and interpreted differently by the different European Member States. Regulatory hurdles must be overcome in a timely manner to obtain approval for clinical trial execution and ultimately marketing authorization. Please consult our regulatory department should you have any questions.
After successful transduction, the CAR-T cells are expanded. The goal of this final step is to increase the number of transduced cells expressing CAR and ultimately obtain the desired amount of cells. For example, young adult patients (50 kg and under) with B-cell ALL receiving Kymriah will require the following dose: 0.2 to 5 x 106 CAR-positive viable T cells/kg body weight. The medicinal product itself will contain 1 or more infusion bags, each containing 1.2 x 106 to 6 x 108 CAR-positive viable T cells. Since millions of cells are needed, yield is a very important factor to consider for these autologous treatments.
Step 5: Isolation of the final product
After expansion, the harvested cells are washed and centrifuged. Cryoprotectants are added for cryopreservation of the drug. The most commonly used method is resuspension in a storage medium containing 10% dimethyl sulfoxide, followed by storage in liquid nitrogen in the vapor phase 10.
Step 6: Administering
Before the product can be administered to the patient via infusion, the patient must undergo conditioning therapy, namely lymphodepletion by chemotherapy.
Challenges of CAR-T cell therapy
Four major challenges will be discussed in this blog post. For more information, please consult QbD’s ATMP experts.
1. Obtaining enough cells
Yield criteria can be very difficult to obtain. Therefore, close interaction with and qualification of the cell supplier (tissue establishment) is crucial to understand the risks of variability of the starting material and to apply a risk-based approach to address these issues during manufacturing. Patient eligibility and medical health status at the time of donation play a crucial role.
2. Safety
CAR-T therapies, while highly effective, are also associated with potentially serious safety complications. Medical personnel should be trained to recognize the symptoms and how to manage them (medication strategies).
- On target/ off-tumor toxicity: If the target antigen is also present in normal tissues, these can also become the target and be destroyed. Therefore, it is crucial to select the right candidate antigen during R&D.
- Cytokine release syndrome (CRS): CRS is caused by the production and release of large amounts of cytokines in the blood from the activated CAR-T cells and host immune cells that will interact with each other. These cytokines can cause symptoms such as fever, severe nausea, hypotension (low blood pressure), hypoxia (low oxygen level in the blood). Severe CRS can lead to organ function impairment or even death.
- Immune effector-associated neurotoxicity syndromes (ICANS): ICANs are associated with increased levels of cytokines in the cerebrospinal fluid due to disruption of the blood-brain barrier. Symptoms such as tremors, seizures, difficulty writing/speaking may occur. Severe conditions can lead to life-threatening cerebral edema.
3. Regulatory framework
Differences in regulatory requirements among European member states (e.g., tissue directives and GMO directives transposed into national legislation) and differences between European and U.S. FDA regulations can be a major obstacle to obtaining clinical trial approval or marketing of products.
4. Commercialization
The manufacturing process, as visualized in Figure 5, is complex and involves difficult supply chain management (e.g., cold chain).
Since these therapies are given to recurrent/refractory patients and not in the first line, the health condition of the patient (donor) is often very severe. Therefore, the time from vein to vein must be kept as short as possible to still be able to administer the product before the patient dies.
As a result of this complex manufacturing/delivery chain and the fact that only one batch of products is made for one patient, the cost of these therapies is very significant. For commercialization, this brings additional uncertainties and reimbursement challenges to make these products commercially available to all. A well-defined health technology assessment should be conducted to evaluate new technological approaches. For more information, contact a Health to Market representative
Future prospects and challenges for CAR-T cell therapy
CAR-T cell therapies have demonstrated their enormous potential in the treatment of CD19 B-cell malignancies. Nevertheless, the anti-tumor activity and safety profile, range of malignancies (such as solid tumors) can be further improved. New strategies to reduce costs and the possibility of switching to off-the-shelf allogeneic products will be further explored.
Some of the obstacles to be overcome in the near future are antigen escape, lack of in vivo persistence, tumor penetration issues, immunosuppressive microenvironment, systematic cytokine toxicity, on-target off-tumor effects and lymphopenia, production time, CAR-T Out of specifications (OOS).
- Regulation (EC) No 1394/2007 of the European Parliament and of the Council of 13 November 2007 on advanced therapy medicinal products and amending Directive 2001/83/EC and Regulation (EC) No 726/2004 (Text with EEA relevance) OJ L 324, 10.12.2007, p. 121–137, CELEX: https://eur-lex.europa.eu/legal-content/EN/TXT/HTML/?uri=CELEX:32007R1394)
- Kuwana, Y., Asakura, Y., Utsunomiya, N., Nakanishi, M., Arata, Y., Itoh, S., Nagase, F., & Kurosawa, Y. (1987). Expression of chimeric receptor composed of immunoglobulin-derived V regions and T-cell receptor-derived C regions. Biochemical and Biophysical Research Communications, 149(3), 960–968.
- Joglekar, A. V., & Li, G. (2021). T cell antigen discovery. Nature Methods, 18(8), 873–880.
- Srivastava, A. D., Unione, L., Bunyatov, M., Gagarinov, I. A., Delgado, S., Abrescia, N., Ardá, A., & Boons, G. J. (2021). Chemoenzymatic Synthesis of Complex N-Glycans of the Parasite S. mansoni to Examine the Importance of Epitope Presentation on DC-SIGN recognition. Angewandte Chemie (International ed. in English), 60(35), 19287–19296.
- Hughes-Parry, H. E., Cross, R. S., & Jenkins, M. R. (2019). The Evolving Protein Engineering in the Design of Chimeric Antigen Receptor T Cells. International Journal of Molecular Sciences, 21(1), 204.
- Singh, A. K., & McGuirk, J. P. (2020). CAR T cells: continuation in a revolution of immunotherapy. The Lancet. Oncology, 21(3), e168–e178
- Han, S., Latchoumanin, O., Wu, G., Zhou, G., Hebbard, L., George, J., & Qiao, L. (2017). Recent clinical trials utilizing chimeric antigen receptor T cells therapies against solid tumors. Cancer letters, 390, 188–200
- Hucks, G., Rheingold, S.R. The journey to CAR T cell therapy: the pediatric and young adult experience with relapsed or refractory B-ALL. Blood Cancer Journal 9, 10 (2019)
- Directive 2004/23/EC of the European Parliament and of the Council of 31 March 2004 on setting standards of quality and safety for the donation, procurement, testing, processing, preservation, storage and distribution of human tissues and cells (OJ L 102 31.03.2004, p. 48, CELEX: https://eur-lex.europa.eu/legal-content/EN/TXT/?uri=CELEX:32004L0023)
- Gee A. P. (2018). GMP CAR-T cell production. Best practice & research. Clinical haematology, 31(2), 126–134.
- Part IV of the Good Manufacturing Practice Guidelines for advanced therapy medicinal products (2017), published in Volume 4 of Eudralex
- Rafiq, S., Hackett, C. S., & Brentjens, R. J. (2020). Engineering strategies to overcome the current roadblocks in CAR T cell therapy. Nature reviews. Clinical oncology, 17(3), 147–167.
- Regulation (EC) No 1394/2007 of the European Parliament and of the Council of 13 November 2007 on advanced therapy medicinal products and amending Directive 2001/83/EC and Regulation (EC) No 726/2004 (Text with EEA relevance) OJ L 324, 10.12.2007, p. 121–137, CELEX: https://eur-lex.europa.eu/legal-content/EN/TXT/HTML/?uri=CELEX:32007R1394)
- Kuwana, Y., Asakura, Y., Utsunomiya, N., Nakanishi, M., Arata, Y., Itoh, S., Nagase, F., & Kurosawa, Y. (1987). Expression of chimeric receptor composed of immunoglobulin-derived V regions and T-cell receptor-derived C regions. Biochemical and Biophysical Research Communications, 149(3), 960–968.
- Joglekar, A. V., & Li, G. (2021). T cell antigen discovery. Nature Methods, 18(8), 873–880.
- Srivastava, A. D., Unione, L., Bunyatov, M., Gagarinov, I. A., Delgado, S., Abrescia, N., Ardá, A., & Boons, G. J. (2021). Chemoenzymatic Synthesis of Complex N-Glycans of the Parasite S. mansoni to Examine the Importance of Epitope Presentation on DC-SIGN recognition. Angewandte Chemie (International ed. in English), 60(35), 19287–19296.
- Hughes-Parry, H. E., Cross, R. S., & Jenkins, M. R. (2019). The Evolving Protein Engineering in the Design of Chimeric Antigen Receptor T Cells. International Journal of Molecular Sciences, 21(1), 204.
- Singh, A. K., & McGuirk, J. P. (2020). CAR T cells: continuation in a revolution of immunotherapy. The Lancet. Oncology, 21(3), e168–e178
- Han, S., Latchoumanin, O., Wu, G., Zhou, G., Hebbard, L., George, J., & Qiao, L. (2017). Recent clinical trials utilizing chimeric antigen receptor T cells therapies against solid tumors. Cancer letters, 390, 188–200
- Hucks, G., Rheingold, S.R. The journey to CAR T cell therapy: the pediatric and young adult experience with relapsed or refractory B-ALL. Blood Cancer Journal 9, 10 (2019)
- Directive 2004/23/EC of the European Parliament and of the Council of 31 March 2004 on setting standards of quality and safety for the donation, procurement, testing, processing, preservation, storage and distribution of human tissues and cells (OJ L 102 31.03.2004, p. 48, CELEX: https://eur-lex.europa.eu/legal-content/EN/TXT/?uri=CELEX:32004L0023)
- Gee A. P. (2018). GMP CAR-T cell production. Best practice & research. Clinical haematology, 31(2), 126–134.
- Part IV of the Good Manufacturing Practice Guidelines for advanced therapy medicinal products (2017), published in Volume 4 of Eudralex
- Rafiq, S., Hackett, C. S., & Brentjens, R. J. (2020). Engineering strategies to overcome the current roadblocks in CAR T cell therapy. Nature reviews. Clinical oncology, 17(3), 147–167.